Research Article - Onkologia i Radioterapia ( 2022) Volume 16, Issue 1
Study of lung respiratory motion influence in patients undertake external beam radiotherapy
Yousif Abdallah1,2*, Nouf Abuhadi3, Elabbas Ebaid4, Bahaeldin Atta5, Abdulrahman M. Alzandi6 and Tariq Alqahtani72Department of Radiotherapy and Nuclear Medicine, College of Medical Radiological Science, Sudan University of Science and Technology, Albaladia Street, Khartoum, Sudan
3Department of Diagnostic Radiographic Technology, College of Applied Medical Science, Jazan University, Jazan, Saudi Arabia
4Department of Radiology, College of Medicine, Majmaah University, Al-Majmaah, Saudi Arabia
5Department of Otolaryngology, College of Medicine, Majmaah University, Al-Majmaah, Saudi Arabia
6Department of Radiological Science, College of Applied Medical Science, Imam Abdulrahman Bin Faisal University, AD Dammam, Saudi Arabia
7Department of Medical Equipment Technology, College of Applied Medical Science, Majmaah University, Al-Majmaah, 11952, Saudi Arabia
Yousif Abdallah, Department of Radiological Science and Medical Imaging, College of Applied Medical Science, Majmaah University, Al-Majmaah, 11952, Saudi Arabia and Department of Radiotherapy and Nuclear Medicine , College of Medical Radiological Science, Sudan University of Science and technology, Albaladia Street, Khartoum, 11111, Sudan, Email: y.yousif@mu.edu.sa
Received: 13-Nov-2021, Manuscript No. M-47323; Accepted: 10-Dec-2021, Pre QC No. P-47323; Editor assigned: 15-Sep-2021, Pre QC No. P-47323; Reviewed: 01-Dec-2021, QC No. QC No. Q-47323; Revised: 10-Dec-2021, Manuscript No. R-47323; Published: 21-Jan-2022
Abstract
Objectives: The aim of this study is to investigate how respiration influences the motion of lung and pancreas tumours and to relate the observations to treatment procedures intended to improve dose alignment by predicting the moving tumour’s position from external breathing indicators. Methods: Breathing characteristics for five healthy subjects were observed by optically tracking the displacement of the chest and abdomen, and by measuring tidal air volume with a spirometer. Fluoroscopic imaging of five radiotherapy patients detected the motion of lung and pancreas tumours synchronously with external and internal breathing indicators. Results: The mean age of the patients in this study was 54.6+9.1 years. 70% of the patients were males and 30% were females. The external and fluoroscopic data showed a wide range of behaviour in the normal breathing pattern and its effects on the position of lung and pancreas tumours. This included transient phase shifts between two different external measures of breathing that diminished to zero over a period of minutes, modulated phase shifts between tumour and chest wall motion, and other complex phenomena. The lung tumour-motion results in this study were 4.8(0-9), 2.9(1.3-6.0), 3.8(1.7-8.2) mm for SI, AP and LR direction, respectively. Conclusion: Respiratory compensation strategies that infer tumour position from external breathing signals, including methods of beam gating and dynamic beam tracking, require three-dimensional knowledge of the tumour’s motion trajectory as well as the ability to detect and adapt to transient and continuously changing characteristics of respiratory motion during treatment
Keywords
lung motion, external beam, radiotherapy
Introduction
Intrafraction mobility has become a significant concern since the introduction of image-guided radiation. An intrafraction motion may be caused by the respiratory, skeletal, cardiac, or gastrointestinal systems. In all three systems, the most recent progress in respiratory motion accounting has been the primary focus of research and development. This presentation will cover radiation oncology in great detail [1-4] (Figure 1).
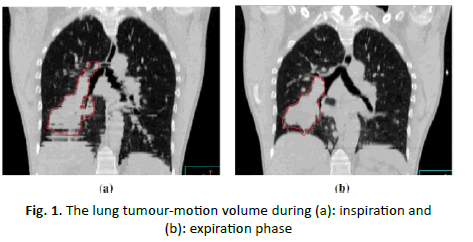
Figure 1: The lung tumour-motion volume during (a): inspiration and (b): expiration phase
Respiratory motion is merely one of many early-process sources of radiation inaccuracy to be considered. There may also be conflicts over the definitions of Gross Tumour Volume (GTV) and Clinical Target Volume (CTV), among other things (CTV). Patients with lung cancer and breast cancer had different GTV and CTV, according to researchers [5-6]. When compared to breathing-related errors, these defects tend to be outweighed. Lung [3, 7-13] and breast [14-21] cancers have setup errors that are comparable to or larger than those associated with respiratory motion setup faults. Artifacts are created when respiratory motion is not taken into account during the picturetaking process, exactly like they are with traditional thoracic and abdominal radiation operations. Depending on the degree of the aberration, it's possible that the final volume will be off by a significant amount [22-33]. CT slice acquisition causes motion artifacts because different regions of the item are shifted in and out of the CT slice view. In CT scans of the thoracic cavity, motion artifacts are common. Figure 2 shows a comparison of Artifacts in CT scans make it difficult to distinguish between target and non-target tissue, making it impossible to produce accurate dosage predictions [25]. The margin of error must be large enough to guarantee that the target is covered for the majority of the therapeutic dose [26-28]. The GTV is commonly defined for CT-guided lung cancer therapy, with a buffer provided to accommodate for the microscopic spread of the disease (which added to the GTV creates the CTV). The International Commission on Radiological Units nomenclature specifies margins for intrafraction and interfraction mobility (due to respiration) and set-up error for computing anticipated target volume. Increasing the amount of healthy tissue exposed all the time and widening treatment borders so that the tumour cannot move are both bad ways of adapting for respiratory mobility. One can clearly see a correlation between the number of patients being treated and the risk of getting complications from their care. As a result, a portion of CTV will be underdosed if the margins are too tiny. It's challenging to determine the proper margin size for accounting for respiratory motion, given the anomalies in CT images that can be seen when that motion isn't taken into account [29].

Figure 2: The chest motion during the respiratory (a) during inhalation phase and (b) AP and LR chest movement
When radiation is delivered to an organ that is moving within a fraction of a second, the dose distribution is averaged or distributed across the path of motion. As a result of this displacement, the desired dose is different from the dose delivered. When a static beam is used, both internal and external displacements are used to calculate the overall positional error affecting the dose in the treatment room. Despite the fact that the dose gradient in the centre of each field is minimized in conventional (non-IMRT) treatments, a blurring of the dose distribution is observed as a result of anatomy migrating along beam edges, effectively expanding the beam penumbra, as illustrated in the figure below. Leaf interactions with target motion perpendicular to the beam are anticipated to exacerbate this effect during IMRT delivery [30]. Multileaf collimators* are anticipated to amplify this effect. This impact was proved using theoretical models, which led to dose adjustments of up to 100% for ‘clinically significant parameters’. In their investigation, Recent research [31-41] demonstrates that, despite the presence of variances in individual fractions, these over-and under dosage variations tend to average out in multifield and multi fraction treatments, resulting in the same averaging artifacts as with conventional treatment regimens. Breathing activates a variety of organs and systems. The impact of this mobility on CT and magnetic resonance pictures, as well as the planning and delivery of radiation doses, has spurred physicists and doctors to investigate it using a variety of imaging modalities [42-50].
Materials and Methods
A sample of 80 patients was scanned using slow-motion CT scanning. We considered previously published data on the effect of breathing on organ motion, among other factors, in this work. Despite the survey's limitations, it may provide patients with some ideas for coping with treatment-related mobility constraints. All of the internal organs of the body move in response to the inhalation and exhalation movements. Researchers in medical physics and clinical medicine have been driven to investigate this motion using a number of imaging modalities due to its effect on the quality of CT and Magnetic Resonance Imaging (MRI) images, as well as on the planning and administration of radiation doses. The effects of breathing and other variables on organ motion are covered throughout this work. Despite the survey's limitations, it may provide you with some ideas for coping with treatment-related mobility constraints. A displacement of up to two millimeters in the Anterior-Posterior (AP) and Right-to-Left (RL) axes is considered usual for abdominal organs. On the other hand, the patterning of other people's kidneys is more intricate. Lung malignancies migrate in a much more diverse manner than other tumours. The rate at which a lung tumour spreads varies significantly according to its location. In this study, the lung cancer patients will study had stationary or incapable of migrating tumours. The IS, AP and LR ranges of motion will be studied [51-59].
Results
Table 1 shows motion measurement techniques. Many authors [44, 46] measured the lung tumour-motion using CT slows for diaphragm. While this study and another authors [38, 43-46, 53-55] studied lung tumor-motion using CT scanning. Slow scanning is a technology that produces extremely precise CT pictures of the body. If the CT scanner is operated at a very slow pace or a large number of scans are averaged, various breathing durations for each slice can be acquired using the slow scanning technique. This means that by scanning the tumour with an X-ray scanner, a precise picture of respiratory function can be obtained (at least in the high contrast areas). Nonetheless, due to the fact that respiratory motion fluctuates between imaging and therapy, additional margins must be introduced to account for these variations. Additional advantages of slow scanning over regular scanning include greater anatomic delineation and dosage estimate, which are achieved by utilizing geometry that more accurately simulates the full breathing cycle during therapy. A single CT scan is possible since the treatment is slowed down by the use of low-resolution CT scanning. Table 1 and Table 2 show lung tumour-motion measurement techniques. The flowchart depicted in Figure 3 should be utilized to treat patients experiencing respiratory issues. Figure 3 illustrates Box 1 inquires whether or not motion can be quantified. It is critical to monitor 3D tumour migration when planning and implementing lung cancer treatment options. To determine tumour mobility, a series of breathing cycles should be done while monitoring the tumour’s movement. Due to the fact that breathing patterns change with age, it is critical to be aware of these changes in the breathing pattern (Table 3).
Site | Technique | |||||
---|---|---|---|---|---|---|
CT scan | MRI | Fluoroscopy | Ultrasound | Nuclear medicine Imaging | Electronic portal imaging device | |
Diaphragm | Giraud et al. [44] | Koria et al. [59] | Wade et al. [46] | Davies et al. [59] | -- | -- |
Ford et al. [51] | ||||||
Lung | This study | Plathow et al [57] | Shimizu et al. [39] | -- | -- | Erridge et al. [56] |
Shimizu et al. [38] Rose et al. [42] Hanley et al. [43] Grills et al. [54] Sixel et al. [55] | Seppenwoolde et al. [40] | |||||
Murphy et al. [47] | ||||||
Chen et al. [49] | ||||||
Barnes et al. [50] | ||||||
Kubo et al. [52] | ||||||
Shirato et al. [53] | ||||||
Ozhasoglu et al. [54] |
Tab. 1. Motion measurement techniques
Observer | 3D motion Measure | ||
---|---|---|---|
SI | AP | LR | |
This study | 4.8 (0-9) | 2.9 (1.3-6.0) | 3.8 (1.7-8.2) |
Grills et al. [54] | Feb-30 | 0-10 | 0-6 |
Erridge et al. [56] | 12.5 (6-34) | 9.4 (5-22) | 7.3 (3-12) |
Ekberg et al. [57] | 3.9 (0-12) | 2.4 (0-5) | 2.4 (0-5) |
Seppenwoolde et al. [40] | 5.8 (0-25) | 2.8 (1.2-5.1) | 3.4 (1.3-5.3) |
Hanley et al. [43] | 12 (1-20) | 5 (0-13) | 1 (0-1) |
Sixel et al. [55] | 0-13 | 0-5 | 0-4 |
Plathow et al. [57] | 9.5 (4.5-16.4) | 6.1 (2.5-9.8) | 6.0 (2.9-9.8) |
Lower lobe | |||
Plathow [57] | 7.2 (4.3-10.2) | 4.3 (1.9-7.5) | 4.3 (1.5-7.1) |
Middle lobe | |||
Plathow [57] | 4.3 (2.6-7.1) | 2.8 (1.2-5.1) | 3.4 (1.3-5.3) |
Upper lobe |
Tab. 2. Lung tumour motion measured data (3D motion measures SI, AP, LR)
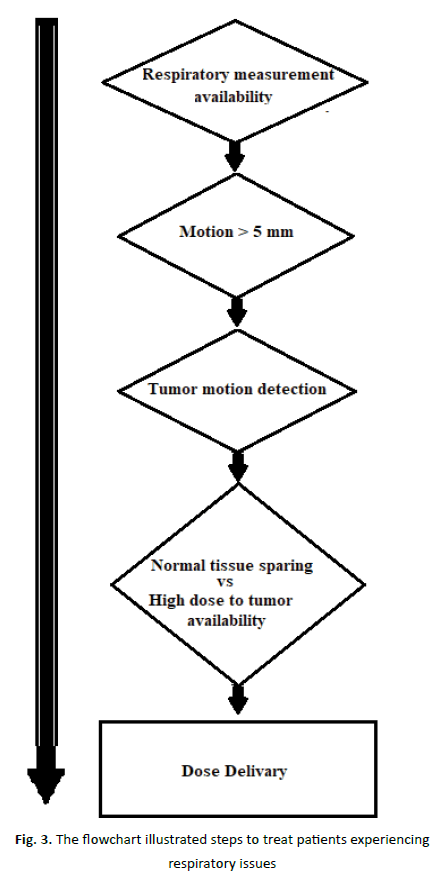
Figure 3: The flowchart illustrated steps to treat patients experiencing respiratory issues
Organ | Respiratory signal | No. of patients | Correlation range | Phase shift | Source |
---|---|---|---|---|---|
Lung tumor, respiratory-correlated CT | Diaphragm position and Abdominal displacement | 80 | 0.43-0.78 | Not observed | This study |
Diaphragm SI | Abdominal displacement | 5(60) | 0.82-0.95 | Not observed | Wade et al. [46] |
Tumor diaphragm, fluoroscopy | Abdominal displacement | 43 | 0.41-0.94 | Short delays observed | |
Tumor, SI fluoroscopy | Spirometry and Abdominal displacement | 11 (23) | 0.39-0.99 | -0.65-5-0.58 | |
Tumor, 3D biplane radiography | Abdominal displacement | 26 | Respiratory waveform cycle agreed with SI and AP tumor motion | Princepally with 0-0.3 Existence of >1.0 |
|
Lung tumor, respiratory-correlated CT | Abdominal displacement | 9 where tumor SI motion>5 mm | 0.74-0.98 | < 1 s 4 ptaients <0.5 s 5 patients |
Mageras et al. [59] |
Lung tumor, SI respiration-correlated CT | Diaphragm position | 12 | 0.74-0.98 | < 1 s 4 patients < 0.5 s 5 patients |
Mageras et al. [59] |
Tab. 3. Correlation of tumour/organ motion with respiratory signal
Discussion
Breath-hold and respiration gated CT scanning can reduce image distortion caused by respiration [13-15, 23]. However, whether the resulting scans are representative of the lesion's location during treatment is an important consideration. Freebreathing scans have minimal distortion on average, which is why they are frequently used for treatment planning. A gated CT scan, on the other hand, is frequently performed after the patient has exhaled, when the lesion speed is at its slowest. If this location is not taken into account, it deviates from the mean tumour position, resulting in a systematic inaccuracy in the patient group. Voluntary breath-hold CT scans are significantly worse than free breathing because deep breathing causes larger displacements than free breathing, potentially introducing extremely significant systematic inaccuracies. Active breathing control allows respiration to be stopped at the average tumour position, but when used for imaging alone, care must be taken to ensure that the respiration pattern does not diverge from free breathing [16-17]. While a patient is still breathing, it is possible to get high-quality CT data utilizing respirationcorrelated CT, commonly known as 4D CT, a technique that records data while the patient is still breathing. 4D data can be combined with additional data to build a 3D model of the average tumour position, the tumour’s range of motion, and its actual mobility. Breathing patterns have a major impact on the acquisition process of 4D CT, which is problematic. Breathing exercises can now be performed in a variety of methods to suit the personal preference. Despite these adjustments, artifacts may still be seen [6-4]. Due to any geometrical flaws in the dosage distribution, the target's position relative to it is shifted. By making the assumptions of a static target and mobile dosage dispersion, it is possible to add the total dose delivered over all fractions in a single computation. Uncertainty about the dosage distribution can emerge when a large number of fractions are present, as is the case when a large number of random errors are present. The following equation defines a convolution of the dosage distribution and the total displacement vector of the target vs. treatment machine, with the treatment machine as the input: The term "blurring" refers to the following: While convolution is a highly reliable technique in practice, it is not always accurate at catching dose changes [26-27]. As a result, systemic weaknesses are overlooked. There are two timedependent actions: breathing and pulse. Unknown random functions are utilized to create the results for adjusting the average breathing depth. The subject is discussed in detail in the article In vivo validation of Elekta’s clarity auto scan for ultrasound-based intrafraction motion estimation of the prostate during radiation therapy [37]. Variations in the sound are caused by the hollow organ's variable loudness. They may be small enough to fit into the lungs or upper abdomen. Almost undoubtedly It is not uncommon for a patient setup error of less than three millimeters to occur (1SD) [11, 28]. Consider the possibility that gated radiation or breath-hold therapies could have an effect on not only the precision of target localization but also on the preservation of normal tissues during treatment. Bear in mind that radiation to the lungs typically causes fast tumour shrinking, complicating drug administration. Bear in mind that planning opacities exist. CT is a significant type of systematic error that should be avoided while developing your project's margin of safety. There are numerous methods for monitoring the patient's breathing motions during radiation treatment. To mention a few, the four primary procedures that account for respiratory motion in radiation include respiratory gate techniques, breath-holds, forced shallow breathing, and breathing synchronized techniques. The patient's breathing cycle is monitored throughout imaging or therapy, and radiation is typically delivered at predefined time intervals (referred to as the "gate" in respiratory gating terminology). The position and width of the gate are controlled during a breathing cycle by either an external respiration signal or internal fiducial markers, depending on the situation. Duty cycle is one of the efficiency metrics. It is estimated by dividing the time spent by the signal inside the "gate" by the overall duration of the therapy. At the time, only the Varian RPM respiratory gating system is available. The technique begins by inhaling a deep breath and holding it for a few seconds. It is likely that deep inspiration breath-hold therapy (sometimes referred to as deep inspiration breath-hold therapy) will prove effective in the treatment of thoracic malignancies since it inhibits tumour migration while protecting critical normal tissues. This study examines a variety of various DIBH implementation strategies. If the patient accepts and actively participates in the treatment, it will be more effective. For Non-Small Cell Lung Cancer (NSCLC), researchers at Memorial Sloan-Kettering Cancer Center (MSKCC) evaluated the efficacy of conformal radiation therapy using a spirometer (MSKCC). When patients use ABC (Active Breathing Control), they can hold their breath for an extended period of time without exhausting their inspiratory capacity [56]. Elekta, Inc. acquired the Active Breathing Coordinator (ABC) technology developed by William Beaumont Hospital, which is now used in hospitals worldwide. If necessary, the ABC device can be used to halt the supply of oxygen at any time throughout the usual breathing cycle. This device, which combines a spirometer and a balloon valve, is used to monitor a patient's breathing patterns. ABC techniques need the patient to utilize a breathing equipment to maintain a constant breathing pattern. Prior to clicking the button to close the balloon, you must first select the volume and phase (inhalation/excretion) of the balloon. The patient is then encouraged to take two preparation breaths to ensure they have adequate lung capacity. An air compressor is used to inflate the patient's airway valve for a predetermined period of time before being shut off. Patients should be able to maintain repeated breath-holds (after a brief rest period) without experiencing discomfort. With the Elekta ABC system, the digital display indicates in green the lung capacity at which the balloon valve will close, allowing for prolonged breath-holding. When a patient is instructed to hold their breath, the term "Self- Held Breath Hold Techniques" is used to describe what occurs in their body. The patient holds their breath as the beam is engaged and a dose is delivered to the tumour. Depending on the area, the length of time a patient must be able to maintain a stable breathing pattern before receiving the correct dose varies. A high-quality accelerator control system is essential for the successful gating of a radiation beam into the breath-hold. The Varian Linac approach makes use of the "Customer Minor (CMNR) interlock" of the Varian C-Series accelerators (Varian Medical Systems, Palo Alto, Ca). The patient triggers the CMNR interlock in this system by pressing a button on a hand-held switch. The switch enables the therapist to operate the beam by disabling the console's CMNR interlock. When the switch is depressed, it activates, preventing further delivery until the switch is depressed again, and it remains activated until the switch is depressed again. The decision to initiate therapy is made together by the therapist and patient. Because the beam delivery system and other safety components already have interlock circuitry, no modifications to the accelerator are required. A comprehensive set of instructions is available in the section under "Treatment." Clinical studies have revealed no statistically significant difference between euphoric and exhausted states of consciousness. This, together with the possible dosimetric benefits of increased lung volume, resulted in the deep inspiration posture being classified as the optimal breath-hold posture for the majority of persons. Pose in a way that gives you a sense of self-worth. The benefits of DIBH are identical to those of the other gating mechanisms discussed before in this section. To be effective, patients must manually hold their breath during the respiratory cycle for a set period of time. Continuous radiation delivery, as opposed to free breathing systems, enables far faster simulations and treatments than with free breathing systems. With 600 micrograms per Minute (MU) inhalation rate, you may deliver 100 micrograms in a ten-second breath hold. When both methods are applied, a free breathing technique can provide the same amount of energy in 30 seconds as a training technique with the same duty cycle and 600 MU per minute. Numerous tracking devices can be used to monitor the patient's breath-hold, with an automated beam hold condition being established if the patient is unable to keep their breath for the required therapy intervals. The author (C) discusses the technique of sighing breathing. At Stockholm's Karolinska Hospital, Lax and Blomgren developed FSB for stereotactic irradiation of small lung and liver lesions. [37, 49] These strategies have been shown to significantly reduce intrafractional mobility [46, 50, 52]. One option is to employ a stereotactic body frame in conjunction with an abdominal pressure plate. While normal breathing can continue, it is constrained by abdominal pressure, which limits diaphragmatic motion and, as a result, gas exchange during the process. Elekta Instruments, Inc. designs and manufactures a number of products, including the body frame and pressure plate system. The most widely accepted technique for compensating for respiratory motion is to dynamically modify the dose in space in response to the tumour’s position moving during free breathing. However, it has been tried solely in a laboratory setting. In the scientific literature, the terms "real-time" or "respiratorysynchronized tracking" are used to describe this technology. Under ideal conditions, a duty cycle of 100% is required to provide appropriate doses, and continuous real-time tracking totally removes the tumour mobility margin. The ability to properly find the tumour in real time, anticipate tumour mobility, adjust the beam, and optimize the dosimetry to account for changes in lung capacity and important anatomical placements during the breathing cycle are all essential for the process to be successful. Gating systems create dynamic feedback by coupling the signal from a respiration-monitoring device to the target's interior position. Due to the dynamic nature of a gating system, it is impossible to examine it using static test phantoms and procedures. To evaluate in vivo dosimetry and target placement using gating systems, dynamic test phantoms that replicate breathing must be used. Clinical respiratory gating therapy systems must undergo acceptance testing, commissioning, and on-going quality assurance using these test phantoms to assure their effectiveness. A three-liter syringe with flow rates ranging from 0.5 L/s to 3 L/s is used in conjunction with the spirometer for calibration. The spirometer's linearity with respect to the actual (syringe) volume is determined throughout a range of 0 L to 3 L in each direction of flow; the average linearity is within 2% of the actual (syringe) volume. Every two months, the calibration is verified for consistency. ABC and DIBH patients are more concerned with the consistency of their breathing and the duration of their breath holds. Preliminary trials revealed a distinction between shortand long-term repeatability, emphasizing the significance of combining ABC with routine image-guided setup adjustments. Before you may utilize this system, you must become familiar with its functionality. It is necessary to document and test breath-hold strategies for specific states (exhale, inhalation, deep inhalation, etc.). To execute breath-holds on a diverse spectrum of persons in varying states of health, specific protocols and documentation must be followed. It is recommended that patients follow a consistent set of patient instructions in order to interact with the ABC operator and undertake emergency breathing re-establishment tasks. All ancillary components must be tracked and maintained. Understanding how consumable items (nose clips, filters, and gas canisters) are used and how reusable items (e.g., rubber mouthpieces) are cleaned is crucial for designing an acceptable set of usage and maintenance protocols for these devices.
Conclusion
Continuous real-time beam tracking is still in its infancy, having been employed in only a few of clinical trials to date. As a result, the necessary quality control methods are being created at the moment. Calibration of both the tumour’s position over time and the spatial link between the tracking and beam delivery coordinate systems is required to address two critical sources of dose delivery error: the tumour’s position and the spatial link between the tracking and beam delivery coordinate systems. Suggestions to Strengthen the Clinician-Client Relationship VII. Specifically, as indicated in Section V, if motion cannot be quantified using a traditional respiratory gated CT technique, the patient should be treated with breathing control. There are two reasons why fluoroscopy (box 1), a widely available motion-measurement technique, may be appealing. To begin, it is affordable. When the motion is negligible in contrast to other radiation faults (as defined in the following paragraph), respiratory control measures are not necessary (box 2). Second, for treatment planning purposes, a patient-specific tumour mobility assessment can and should be incorporated into the patient's CTV to PTV margin. When determining a patient's internal margin, it is crucial to consider the patient's whole range of motion. If respiratory control devices are used, only the patient's lungs' motion should be considered during radiation treatment administration. Treatment visits are organized on a regular basis. It is advised that while determining CTV-PTV margins for treatment planning, the following respiratory motion aspects be considered: By using a surrogate structure, such as the chest wall or diaphragm, to track or "breath-hold" the tumour during treatment, these fears can be alleviated without the patient needing to be present to witness it first-hand. Two more difficulties that arise across all regions are setup errors and tumour alterations following radiation treatment. Due to the lack of quantification, educated guesses are required regarding the amount to which each of the aforementioned defects exists. The following section provides information on research initiatives aimed at closing knowledge gaps. At least one physical scientist must be present during all imaging and treatment sessions, as well as at the start of each patient's therapy, to ensure proper treatment of respiratory motion disorders. A physicist should be accessible for advice throughout the formulation and implementation of the treatment. Each piece of equipment should be demonstrated to the scientists performing the task so that they are familiar with its functionality (s).
Competing Interest
Authors have declared that no competing interests exist.
References
- Acharya S, Fischer-Valuck BW, Kashani R, Parikh P, Yang D, et al. Online magnetic resonance image guided adaptive radiation therapy: first clinical applications. Int J Radiat Oncol Biol Phy. 2016;94:394-403.
- Bainbridge HE, Menten MJ, Fast MF, Nill S, Oelfke U et al. Treating locally advanced lung cancer with a 1.5 T MR-Linac–Effects of the magnetic field and irradiation geometry on conventionally fractionated and isotoxic dose-escalated radiotherapy. Radiat Oncol. 2017;125:280-285.
- Ballhausen H, Li M, Hegemann NS, Ganswindt U, Belka C. Intra-fraction motion of the prostate is a random walk. Phy Med Biol. 2014;60:549.
- Batin E, Depauw N, MacDonald S, Lu HM. Can surface imaging improve the patient setup for proton postmastectomy chest wall irradiation? Prac radiat oncol. 2016;6:e235-e241.
- Bertholet J, Toftegaard J, Hansen R, Worm ES, Wan H et al. Automatic online and real-time tumour motion monitoring during stereotactic liver treatments on a conventional linac by Combined Optical and Sparse Monoscopic Imaging with Kilovoltage x-rays (COSMIK). Phy Med Biol. 2018;63:055012.
- Bertholet J, Wan H, Toftegaard J, Schmidt ML, Chotard F, Parikh PJ et al. Fully automatic segmentation of arbitrarily shaped fiducial markers in cone-beam CT projections. Phy Med Biol. 2017;62:1327.
- Bertholet J, Worm ES, Fledelius W, Høyer M, Poulsen PR. Time-resolved intrafraction target translations and rotations during stereotactic liver radiation therapy: implications for marker-based localization accuracy. Int J Radiat Oncol Biol Phy. 2016;95:802-809.
- Boda-Heggemann J, Weiss C, Vogel L, Siebenlist K, Sihono DS et al. Ultrasound-based real-time tracking during abdominal stereotactic body radiation therapy: ultrasound probe does not influence plan quality significantly. J Radiat Oncol Biol Phy. 2016;96:E604-E605.
- Booth JT, Caillet V, Hardcastle N, O’Brien R, Szymura K et al. The first patient treatment of electromagnetic-guided real time adaptive radiotherapy using MLC tracking for lung SABR. Radiat Oncol. 2016;121:19-25.
- Bourque AE, Bedwani S, Filion É, Carrier JF. A particle filter based autocontouring algorithm for lung tumour tracking using dynamic magnetic resonance imaging. Med phy. 2016;43:5161-5169.
- Braide K, Lindencrona U, Welinder K, Götstedt J, Ståhl I et al. Clinical feasibility and positional stability of an implanted wired transmitter in a novel electromagnetic positioning system for prostate cancer radiotherapy. Radiat Oncol. 2018;128:336-342.
- Campbell WG, Jones BL, Schefter T, Goodman KA, Miften M. An evaluation of motion mitigation techniques for pancreatic SBRT. Radiat Oncol. 2017;124:168-173.
- Campbell WG, Miften M, Jones BL. Automated target tracking in kilovoltage images using dynamic templates of fiducial marker clusters. Med phy. 2017;44:364-374.
- Camps SM, Houben T, Fontanarosa D, Edwards C, Antico M et al. One-class Gaussian process regressor for quality assessment of transperineal ultrasound images. In Proceedings of the 1st International Conference on Medical Imaging with Deep Learning 2018 2018;1-10.
- Camps SM, Verhaegen F, Vanneste BG, de With PH, Fontanarosa D. Automated patient‐specific transperineal ultrasound probe setups for prostate cancer patients undergoing radiotherapy. Med phy. 2018;45:3185-3195.
- Castellanos CB. World‐Systems Theory. Int Encycl Anthropol. 2018;25:1-9.
- Chang JY, Zhang X, Knopf A, Li H, Mori S et al. Consensus guidelines for implementing pencil-beam scanning proton therapy for thoracic malignancies on behalf of the PTCOG thoracic and lymphoma subcommittee. Int J Radiat Oncol Biol Phy. 2017;99:41-50.
- Chi Y, Rezaeian NH, Shen C, Zhou Y, Lu W, Yang M, Hannan R, Jia X. A new method to reconstruct intra-fractional prostate motion in volumetric modulated arc therapy. Phy Med Biol. 2017;62:5509.
- Cho J, Cheon W, Ahn S, Jung H, Sheen H et al. Development of a real-time internal and external marker tracking system for particle therapy: a phantom study using patient tumour trajectory data. J Radiat Res. 2017 Sep 1;58(5):710-719.
- Chung H, Poulsen PR, Keall PJ, Cho S, Cho B. Reconstruction of implanted marker trajectories from cone‐beam CT projection images using interdimensional correlation modeling. Med phy. 2016;43:4643-4654.
- Ciocca M, Mirandola A, Molinelli S, Russo S, Mastella E et al. Commissioning of the 4-D treatment delivery ssystem for organ motion management in synchrotron-based scanning ion beams. Phy Med. 2016;32:1667-1671.
- Datta A, Aznar MC, Dubec M, Parker GJ, O'Connor JP. Delivering functional imaging on the MRI-linac: current challenges and potential solutions. Clin Oncol. 2018;30:702-710.
- De Luca V, Banerjee J, Hallack A, Kondo S, Makhinya M et al. Evaluation of 2D and 3D ultrasound tracking algorithms and impact on ultrasound‐guided liver radiotherapy margins. Med phy. 2018;45:4986-5003.
- Delcoudert L, Fargier-Voiron M, Munoz A, Pommier P, Biston MC. Covalidation of the performances of a transperineal ultrasound probe and electromagnetic transmitter for monitoring prostate cancer motions in radiotherapy. Physica Medica: Eur J Med Phy. 2017;44:4-5.
- Dhont J, Vandemeulebroucke J, Burghelea M, Poels K, Depuydt T et al. The long-and short-term variability of breathing induced tumour motion in lung and liver over the course of a radiotherapy treatment. Radiat Oncol. 2018;126:339-346.
- Ehrbar S, Schmid S, Jöhl A, Klöck S, Guckenberger M et al. Comparison of multi-leaf collimator tracking and treatment-couch tracking during stereotactic body radiation therapy of prostate cancer. Radiat Oncol. 2017;125:445-452.
- Ehrbar S, Schmid S, Jöhl A, Klöck S, Guckenberger M et al. Validation of dynamic treatment‐couch tracking for prostate SBRT. Med phy. 2017;44:2466-2477.
- Fassi A, Ivaldi GB, de Fatis PT, Liotta M, Meaglia I et al. Target position reproducibility in left‐breast irradiation with deep inspiration breath‐hold using multiple optical surface control points. J Appl Clin Med Phys. 2018;19:35-43.
- Fattori G, Safai S, Carmona PF, Peroni M, Perrin R et al. Monitoring of breathing motion in image-guided PBS proton therapy: comparative analysis of optical and electromagnetic technologies. Radiat Oncol. 2017;12:1-1.
- Feng Y, Kawrakow I, Olsen J, Parikh PJ, Noel C et al. A comparative study of automatic image segmentation algorithms for target tracking in MR‐IGRT. J Appl Clin Med Phys. 2016;17:441-460.
- Fledelius W, Keall PJ, Cho B, Yang X, Morf D, Scheib S et al. Tracking latency in image-based dynamic MLC tracking with direct image access. Acta Oncol. 2011;50:952-959.
- Fuchs H, Moser P, Gröschl M, Georg D. Magnetic field effects on particle beams and their implications for dose calculation in MR‐guided particle therapy. Med phy. 2017;44:1149-1156.
- Fukada J, Hanada T, Kawaguchi O, Ohashi T, Takeuchi H, et al. Detection of esophageal fiducial marker displacement during radiation therapy with a 2-dimensional on-board imager: analysis of internal margin for esophageal cancer. Int J Radiat Oncol Biol Phy. 2013;85:991-998.
- Gerlach S, Kuhlemann I, Ernst F, Fürweger C, Schlaefer A. Impact of robotic ultrasound image guidance on plan quality in SBRT of the prostate. British J Radiol. 2017;90:20160926.
- Ginn JS, Agazaryan N, Cao M, Baharom U, Low DA et al. Characterization of spatial distortion in a 0.35 T MRI-guided radiotherapy system. Phy Med Biol. 2017;62:4525.
- Glitzner M, Woodhead PL, Lagendijk JJ, Raaymakers BW. OC-0189: first MLC-tracking on the 1.5 T MR-linac system. Radiat Oncol. 2018 Apr 1;127:S101-2.
- Grimwood A, McNair H A, O’Shea T P, Gilroy S, Thomas K et al. In vivo validation of Elekta’s clarity autoscan for ultrasound-based intrafraction motion estimation of the prostate during radiation therapy. Int J Radiat Oncol Biol Phy. 2018;102:912-921.
- Shimizu S, Shirato H, Kagei K, Nishimura M, Miyasaka K, et al. Detection of lung tumor movement in real-time tumor-tracking radiotherapy. Int J Radiat Oncol Biol Phys. 2001;51:304-310.
- Shimizu S, Shirato H, Kagei K, Nishioka T, Bo X, et al. Impact of respiratory movement on the computed tomographic images of small lung tumors in Three-Dimensional (3D) radiotherapy. Int J Radiat Oncol Biol Phys. 2000;46:1127-1133.
- Seppenwoolde YH, Shirato K, Kitamura S, Shimizu M, Miyasaka K, et al. Precise and real-time measurement of 3D tumor motion in lung due to breathing and heartbeat, measured during radiotherapy. Int J Radiat Oncol Biol Phys. 2002;53:822-834.
- Stevens CW, Munden RF, Forster KM, Kelly JF, Liao Z, et al. Respiratory-driven lung tumor motion is independent of tumor size, tumor location, and pulmonary function. Int J Radiat Oncol Biol Phys. 2001;51:62-68.
- Ross CS, Hussey DH, Pennington EC, Stanford W, Doornbos JF. Analysis of movement of intrathoracic neoplasms using ultrafast computerized tomography. Int J Radiat Oncol Biol Phys. 1990;18:671-677.
- Hanley J, Debois MM, Mah D, Mageras GS, Raben A, et al. Deep inspiration breath-hold technique for lung tumors: The potential value of target immobilization and reduced lung density in dose escalation. Int J Radiat Oncol Biol Phys. 1999;45:603-611.
- Giraud P, Rycke DY, Dubray B, Helfre S, Voican D, et al. Conformal Radiotherapy (CRT) planning for lung cancer: analysis of intrathoracic organ motion during extreme phases of breathing. Int J Radiat Oncol Biol Phys. 2001;51:1081-1092.
- Korin HW, Ehman RL, Riederer SJ, Felmlee JP, Grimm RC. Respiratory kinematics of the upper abdominal organs: a quantitative study.” Magn Reson Med. 1992;23:172-178.
- Wade O. Movement of the thoracic cage and diaphragm in respiration. J Physiol 1954;124:193-212.
- Murphy MJ, Adler JR, Bodduluri M, Dooley J, Forster K, et al. Image-guided radiosurgery for the spine and pancreas. Comput. Aided Surg. 2000;5:278-288.
- Martin MJ, and Poen J, Martin D, Dooley J, Hai J, et al. Image-guided radiosurgery for the spine and pancreas. Comput Aided Surg. 2000;5:278-288.
- Chen QS, Weinhous MS, Deibel FC, Ciezki JP, Macklis RM. et al. Fluoroscopic study of tumor motion due to breathing: Facilitating precise radiation therapy for lung cancer patients. Med Phys. 2000;28:1850-1856.
- Barnes EA, Murray BR, Robinson DM, Underwood LJ, Hanson J, et al. Dosimetric evaluations of lung tumor immobilization using breath hold at deep inspiration. Int J Radiat Oncol Biol Phys. 2001;50:1091-1098.
- Ford EC, Mageras GS, Yorke E, Rosenzweig KE, Wagman R, et al. Evaluation of respiratory movement during gated radiotherapy using film and electronic portal imaging. Int J Radiat Oncol Biol Phys. 2002;52:522-531.
- Weiss PH, Baker JM, Potchen EJ. Assessment of hepatic respiratory excursion. J Nucl Med. 1972;13:758-759.
- Ozhasoglu C, Murphy MJ. Issues in respiratory motion compensation during external-beam radiotherapy. Int J Radiat Oncol Biol Phys. 2002;52:1389-1399.
- Grills IS, Yan D, Martinez AA, Vicini FA, Wong JW, et al. Potential for reduced toxicity and dose escalation in the treatment of inoperable non-small-cell lung cancer: a comparison of Intensity-Modulated Radiation Therapy (IMRT), 3D conformal radiation, and elective nodal irradiation. Int J Radiat Oncol Biol Phys. 2003;57:875-890.
- Sixel KE, Ruschin M, Tirona R, Cheung PC. Digital fluoroscopy to quantify lung tumor motion: potential for patient-specific planning target volumes. Int J Radiat Oncol Biol Phys 2003;57:717-723.
- Erridge SC, Seppenwoolde Y, Muller SH, Herk M, Jaeger KD, et al. Portal imaging to assess set-up errors, tumor motion and tumor shrinkage during conformal radiotherapy of non-small cell lung cancer. Radiother Oncol. 2003;66:75-85.
- Plathow C, Ley S. Fink C, Puderbach M, Hosch W, et al. Analysis of intrathoracic tumor mobility during whole breathing cycle by dynamic MRI. Int J Radiat Oncol Biol Phys. 2004;59:952-959.
- Shirato H, Shimizu S, Kitamura K, Nishioka T, Kagei K, et al. Four-dimensional treatment planning and fluoroscopic real-time tumor tracking radiotherapy for moving tumor. Int J Radiat Oncol Biol Phys. 2000;48:435-442.
- Mageras GS, Yorke E, Jiang S. 4D IMRT Delivery. Image-guided IMRT. 2005;269-285.