Research Article - Onkologia i Radioterapia ( 2023) Volume 17, Issue 9
Dose-response characterization of a prototype aluminium oxide detector for megavoltage photon dosimetry
Hadi Nasrollahi1*, Mohsen Hasani2, Hasan Ali Nedaei2, Hamideh Naderi3 and Hossein Arabi42Department of Radiotherapy Physics, Cancer Research Centre, Cancer Institute, Tehran University of Medical Sciences, Tehran, Iran
3Department of Radiotherapy Physics, Qom University of Medical Sciences, Qom, Iran
4Division of Nuclear Medicine & Molecular Imaging, Geneva University Hospital, CH-1211, Geneva, Swaziland
Hadi Nasrollahi, Department of Radiotherapy Physics, Turkish Hospital, Karbala, Iraq, Email: hnasrollahi@muq.ac.ir
Received: 31-Jul-2023, Manuscript No. OAR-23-108648; Accepted: 07-Sep-2023, Pre QC No. OAR-23-108648 (PQ); Editor assigned: 05-Aug-2023, Pre QC No. OAR-23-108648 (PQ); Reviewed: 25-Aug-2023, QC No. OAR-23-108648 (Q); Revised: 03-Sep-2023, Manuscript No. OAR-23-108648 (R); Published: 15-Sep-2023
Abstract
Background: Aluminium oxide (Al2O3:C), a material with Radio Luminescence (RL) property, is extensively used for dosimetry of ionizing radiations due to its high sensitivity, small size, and low fading properties. The present study aims to investigate the response rate of a prototype aluminium oxide (Al2O3) detector, previously introduced, for megavoltage photon beam dosimetry, and also to determine its dosimetric characteristics. Materials and methods: A piece of the prototype Al2O3 crystal was cut into smaller fragments (0.2 cm3 × 0.2 cm3 × 0.1 cm3). Then, the calibration curve of the dosimeters was obtained through investigating its luminescence feature while exposed to 6 mV, 10 mV, and 18 mV photon beams using an Elekta linear accelerator (linac). The dependence of the dosimeter response on the energy of the radiation beam, dependence of the dosimeter response to the dose alteration, and its repeatability were also investigated. Results: For the megavoltage photon energies, the prototype Al2O3 crystal exhibited dosimetric responses independent of the dose changes and energy of the radiation beam (photons). This dosimeter showed a linear calibration curve with three peaks at 308, 375, and 595 nm, as well as acceptable repeatability in the measurement of radiation doses ranging from 20 cGy to 200 cGy. Conclusion: The prototype Al2O3 crystal exhibited reproducible and reliable dosimetric properties which can be exploited for the dosimetry megavoltage photon beams in radiotherapy clinical settings.
blog
blog
blog
blog
blog
blog
blog
blog
blog
blog
blog
blog
blog
blog
blog
blog
blog
blog
blog
blog
blog
blog
blog
blog
blog
blog
blog
blog
blog
blog
blog
blog
blog
blog
blog
blog
blog
blog
blog
blog
blog
blog
blog
blog
blog
blog
blog
blog
blog
Keywords
Optically Stimulated Luminescence (OSL), radiotherapy, dosimetry, AL2O3 crystal
Introduction
One of the requirements of high-quality radiotherapy is accurate and precise dosimetry. For this purpose, various dosimeters have been introduced/developed commercially and most of them are expensive. In this light, it would be very practical to develop a dosimeter with desired quality and accuracy and low cost.
High sensitivity to a wide range of energies, tissue-equivalency, repeatability of the dosimeter response, high stability, low fading, linear response absorbed dose changes, and independence to irradiation rate are the critical factors for a substance to be used in dosimetry practice [1, 2]. Several dosimeters are currently used for clinical applications in radiotherapy centers, each with specific advantages and disadvantages including.
For instance, EBT (External Beam Therapy) radiochromic film is a tissue-equivalent with high resolution; however, it shows high sensitivity to UV (Ultraviolet) rays [3-5]. Thermoluminescent Dosimeters (TLDs), despite their widespread use in radiotherapy centres, lose part of the dosimetric information within the reading process. The process of fading occurs when a detector's latent information is unintentionally lost, primarily as a result of heat action [6, 7]. Some materials have thermos luminescence properties where part of the waves is usually absorbed after radiating to an object, and then will be emitted in the form of heat. However, in some minerals such as quartz (SiO2 ), fluoroperovskites (NaMgF3 :Eu2+), and Aluminium oxide (Al2O3C), part of the absorbed energy changes into higherwavelength light, and its colour will be changed. This process is called luminescence, which occurs due to electronic transitions after applying physical stimulation [8]. Such luminescent dosimetry is referred to as Optically Stimulated Luminescence (OSL) [9, 10].
In 1987, Al2O3 crystal was used as a dosimeter for measuring doses from ionizing radiation due to its specific model of energy absorption and emission. However, aluminium oxide (Al2O3 :C) has been used more extensively than TLD in dosimetry practices because of its higher sensitivity and lower fading properties [11]. Non-tissue-equivalency is the major defect of this dosimeter compared to TLD. The effective atomic number of Al2O3 crystal is in the range of 10-12, whereas this is about 7 for the body tissue. A correction coefficient is therefore needed in the dosimetry practices, which is often achieved through Monte Carlo simulations [12].
The present study aims to investigate the dosimetric haracteristics of a prototype aluminium oxide (Al2O3 :C) through its luminescence properties in the range of megavoltage photon beams. This prototype Al2O3 :C was developed by Mohammadi. (The Research Center of Malek-e-Ashtar University of Technology (MUT, Tehran, Iran)) wherein its performance was investigated with Elekta precise linac different irradiations [13].
Methods
Al2O3 crystal
The first report on the application of OSL for measuring doses from ionizing radiation in radiotherapy centre’s was presented by Huston et al. [14]. In recent years, Al2O3 crystal has been widely used in radiotherapy centres. This crystal (being used in the form of powder and chips) is sensitive to visible wavelengths ranging from 250 nm to 600 nm [15].
Luminescent materials are capable of absorbing energy, and Al2O3 is one of the most widely used luminescent materials owing to its Optically Stimulated Luminescence (OSL) properties for dosimetry
Figure shows how energy is absorbed and reflected from luminescent materials, where the absorption of energy by electrons creates empty spaces (holes) in the material, and the stimulated electron goes to higher levels. Light is emitted when the electron returns from higher to middle and lower energy levels (Figure 1) [16].
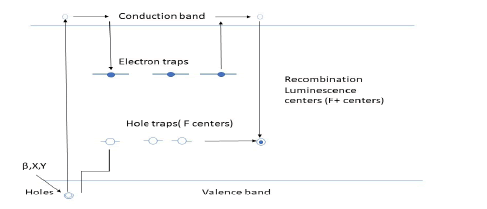
Figure 1: Luminescence is divided into different types, including luorescence, phosphorescence, thermoluminescence, and triboluminescence
Considering the significant increase in the demand for alumina ceramics and especially ÃÃÂ???-Al2O3 , and also the inability of the traditional Bayer method to produce ÃÃÂ???-Al2O3 powders with a high surface area, several chemical methods have been proposed including precipitation, spray pyrolysis, sol-gel, organic precursors, pechini, etc. Nevertheless, these methods require complicated and time-consuming techniques, which will be an obstacle to repeatability, low final price, and high reliability for the final powders [17-22].
The solution to some of the problems mentioned above is The Solution Combustion Synthesis (SCS) method which includes an exothermic reaction between an oxidant such as metal nitrate, ammonium nitrate, and ammonium perchlorate as well as an organic fuel such as urea (CH4N2O), carbohydrazide (CH6N4O) or glycine (2C2H5NO) [23].
Compared to traditional solid-state methods, powders obtained from combustion synthesis are usually more homogeneous,with less impurity and more surface area. The combustion reaction mechanism is relatively complex. The parameters that affect the reaction are fuel type, fuel-to-oxidizer ratio, use of additional oxidizer, combustion temperature, and amount of water in the initial mixture. When the reaction is complete, the only gases produced are 2CO2 , N, and H2O, which makes it an environmentally friendly method.
The most vital advantages of this process are low energy required (no additional annealing is needed), saving time (the entire process takes only a few minutes), and being environmentally friendly (combustion reaction products include: 2CO2 , N, and H2O).
A strategy to increase thermoluminescence properties is to create structural defects in ÃÃÂ???-Al2O3 , which is done by using different impurities as dopants. In this prototype, lithium and copper impurities were applied to improve the thermoluminescence properties of ÃÃÂ???-Al2O3 . We used the SCS method to produce pure ÃÃÂ???-Al2O3 and introduce impurities into the crystal structure, which is a cheap and practical procedure [24].
To prepare non-doped samples, stoichiometric amounts of nonahydrate aluminum nitrate (Al(NO3 )3.9H2 O) and urea (H4N2CO) used as fuel (based on reaction 1) were dissolved in 50 mL of double ionized water with increase the temperature to 200°C and placed on a magnetized heater with a temperature of 50°C and a speed of 360 rpm for 30 minutes. After 30 minutes, the temperature increased to 200°C, and the solution was allowed to dehydrate.
Then, what remained was poured into an aluminium crucible and transferred to the furnace. Then this powder was collected and pounded in a mortar and placed in an aluminium crucible for annealing. 3LiNO and Cu(N03)2.3H2 O were used for doping lithium and copper inside the alumina structure. The weight percentage of impurities used in this study are 0.5, 1, and 3% lithium and 0.1, 0.5, and 1% copper added to alumina. A combination of 1% lithium and 0.5% copper for the joint dope was used as a dopant. Being very high hardness, a crystal was cut by diamond blades into 20 smaller fragments each with (0.2 cm3 × 0.2 cm3 × 0.1 cm3 ). All dosimeters were provided in the same shape and weight, due to the direct effect of the crystal's size and weight on the dosimetric response and sensitivity.
In this project, the prototype Al2O3 crystal developed at the Research Center of Malek-e-Ashtar University of Technology (MUT, Tehran, Iran) was employed. The optimal level of the stimulus wavelength associated with the dosimeter was first obtained. The entire dosimetric parameters of this prototype, including the calibration curve, excitation wavelength, dependence of the dosimeter response on the radiation energy and the dose changes, and its repeatability were investigated. All measurements were carried out at the Alborz Radiotherapy Center (Alborz Province, Iran) by the Elekta precise linac with energies of 6 mV, 10 mV, and 18 mV and a dose rate range of 100 MU/min to 600 MU/min. The RadCalc v5.2 (Lifeline Software, Texas, USA) was used to calculate the amounts of MU that were used at each measurement. Crystal readings were also carried out by the PerkinElmer LS-55 Fluorescence Spectrometer (120 V).
Calibration curve
In this study, 21 chips-liked crystals (0.2 cm3 × 0.2 cm3 × 0.1 cm3 ) were employed and placed at 410°C for 10 minutes to remove the previous effects of radiation on the crystals due to the annealing process. To calculate Element Correction Coefficients (ECC), all crystals were exposed to a 6 mV photon beam and dose of 100 cGy by the Elekta Precise linear accelerator. The irradiation was performed in a Perspex phantom under the condition of a 10 cm2 ×10 cm2 field sizes at 100 cm Source to Surface Distance (SSD) and depth of 2 cm. Five Perspex slabs (each with a thickness of 1 cm) were placed under the crystals to create a complete electron equilibrium condition. The PerkinElmer LS-55 Fluorescence Spectrometer (120 V) was used to read the crystals. After reading the crystals, the ECCs were calculated for each dosimeter using the following relation:
Where (OLR)j is the reading for each dosimeter and OLR is the mean of the total measurements.
After calculating the ECCs, the crystals whose readings were outside the average ± σ range were not used in the project. σ refers to the standard deviation, which is one of the dispersion indicators. Crystal readings were performed in terms of the number of counts. To convert these values to the absorbing doses, the crystals were calibrated in a group against specific doses, and then the calibration curve was plotted. For each dose level, three Al2O3 crystals were irradiated a 10 cm2 × 10 cm2 field and an SSD of 100 cm. Fourteen crystals received doses of 0, 20 cGy, 40 cGy, 70 cGy, 100 cGy, 150 cGy, and 200 cGy. The zero value refers to the background radiation and the calibration curve was plotted in the energy mode of 6 mV using the ECC coefficients.
Evaluation of dosimetric characteristics of the Al2O3 dosimeters
For evaluation of the Al2O3 crystals dosimetric characteristics, the response to different beam energy, dose rate, and reputability was investigated. The evaluation metrics were OSLs responses that were measured with The PerkinElmer LS-55 fluorescence spectrophotometer (120 V). The independence of the dosimeters’ response to the energy of radiation is one of the most important features of a dosimeter. The Elekta's energy modes of 6 mV, 10 mV, and 18 mV were used to assess this issue. The crystals have received a dose of 200 cGy in the above-mentioned energies. After reading, the variation curve of dose-response was plotted against the energy of the radiation beams. In this process, three crystals were used for each irradiation, and the mean readings were considered. Fifteen crystals were used to investigate the response of the crystals to the radiation's dose rate changes. They were all exposed to a photon beam of 6 mV and dose rates of 100 MU/min, 200 MU/min, 300 MU/min, 400 MU/min, 500 MU/min and 600 MU/min by Elekta linac. At each irradiation, three Al2O3 crystals were exposed to a dose of 100 cGy, and the dose rate variation curve was plotted against the dose rate response of radiation beams.
To assess the repeatability of the reading of the absorbing dose, three Al2O3 crystals were exposed to energy of 6 mV and under a dose of 100 cGy. The average reading of three crystals was calculated and recorded. This was repeated four times, and the repeatability curve of the dosimeter response was obtained.
Spectrophotometry
The PerkinElmer LS-55 fluorescence spectrophotometer (120 V) was used to read the Al2O3 chips. The device has the ability to measure fluorescence, phosphorescence, and bioluminescence for liquid, solid, powder, and thin film samples. The device's excitation wavelength is 200 nm-800 nm. A Xenon lamp, which can record pulses of up to 900 nm in milliseconds, was used to create the excitation wavelength.
Results
Earth Continuity Conductor (ECC)
To calculate ECCs, all crystals were irradiated to a photon beam of 6 MV and a dose of 100 cGy by the Elekta linac. The PerkinElmer LS-55 Fluorescence Spectrometer can emit an excitation wavelength from 200 nm-800 nm to stimulate the crystal's energy states. In this study, the optimal wavelength for reading crystals was 260 nm. When the wavelength is more than 260 nm, the crystal is saturated, and it does not provide any specific spectrum. Lower wavelengths cannot stimulate crystal's energy states, and the emitted photons would not produce a specific spectrum. There is a good agreement between the finding in this study regarding the stimulated wavelength and previous studies. The spectrum obtained from emitting a 260 nm wavelength to the Al2O3 crystal is shown in (Figure 2).
Figure 2: The spectrum obtained from the reading of the Al2O3 crystal using the PerkinElmer LS-55 Fluorescence Spectrometer at an excitation wavelength of 260 nm. The spectrum has three peaks: changes in peaks 1 and 2 are significant in numerous readings, whereas the variations of the third peak were negligible
The spectrum obtained from the reading of the Al2O3 crystals has three peaks at 308 nm, 375 nm, and 595 nm (Figure 2). A suitable range of wavelength for Al2O3 OSL stimulation is within the blue and green wavelengths (450-570 nm) [25,26]. In this regard, the third peak at 525 nm-800 nm is used as the light wavelength for the OSL reader. The ECC coefficients were obtained after reading crystals by the PerkinElmer LS-55 Fluorescence Spectrometer at an excitation wavelength of 260 nm using equation 2. The readings of crystals and calculated coefficients are presented in (Table 1).
Tab. 1. Calculated ECCs obtained from the reading of the Al2O3 crystal
Al2O3 number | Al2O3 reading (counts) | ECC | Al2O3 number | Al2O3 reading (counts) | ECC |
---|---|---|---|---|---|
1 | 2.157 × 10 4 | 0.996 | 11 | 2.157 × 104 | 1 |
2 | 2.152 × 104 | 0.998 | 12 | 2.152 × 104 | 0.997 |
3 | 2.149 × 104 | 0.997 | 13 | 2.149 × 104 | 0.996 |
4 | 2.155 × 104 | 1.007 | 14 | 2.155 × 104 | 0.999 |
5 | 2.147 × 104 | 0.998 | 15 | 2.147 × 104 | 0.995 |
6 | 2.153×104 | 0.999 | 16 | 2.153 × 104 | 0.998 |
7 | 2.149 × 104 | 1.001 | 17 | 2.149 × 104 | 0.996 |
8 | 2.157 × 104 | 1.009 | 18 | 2.157×104 | 1 |
9 | 2.161 × 104 | 0.999 | 19 | 2.161 × 104 | 1.002 |
10 | 2.164 × 104 | 1.002 | 20 | 2.164 × 104 | 1.003 |
Average | 0.757 × 104 | 0.757 × 104 |
Regarding the average ± σ criteria, the readings of crystals No. 4, 8, and 15 were out of range (2.149 ×104 , 2.164×104 ) hence, 17 of the 20 crystals were investigated in the study. The dosimeters’ responses were harmonized using the calculated ECCs, and the following results were obtained/reported after harmonization corrections.
In this study, 14 crystals were used to plot the calibration curve, wherein they were exposed to doses of 20 cGy, 40 cGy, 70 cGy, 100 cGy, 150 cGy, and 200 cGy under a standard dosimetric condition (SSD of 100 cm and field size of 10 cm2 × 10 cm2 ). Two crystals were not exposed to the photon beam to register the absorbed dose from the background radiation. The average absorbed dose of these two crystals was considered the calibration factor. The average readings of these two crystals were subtracted from the readings of irradiated crystals (Figure 3).
Figure 3: The Al2O3 crystal's dose-response curve using perspex phantom with a 3 cm build-up cap and photon energy of 6 MV. The dose measurement was within a range of 20 cGy to 200 cGy, under standard dosimetric circumstances
Figure depicts the calibration curve linearly fitted to the measurements (y=0.0044x+1.37) The Elekta linac with energy beams of 6, 10, and 18 mV was employed to assess the dosimetric responses versus the variations of radiation energy. To this end, a 1 cm perspex plate was placed on the dosimeters. The dosimeter was positioned in depth of after the build-up region. The variation curve for the crystal's absorbed dose at the dose of 200 cGy in different energies is shown in (Figure 4). The data obtained from the dosimeter response using the 6 mV beam were then normalized to the max of readings. At this step, 9 crystals were used, and the average value of three crystals was considered as the main reading.
Figure 4: The variation curve of the Al2O3 OSL response relative to energy changes. Energy modes of 6 mV, 10 mV, and 18 mV were considered. The readings for a dose of 200 cGy were normalized to the 6 mV energy reading
Regarding Figure, the absorbed dose of the dosimeter depends, on the beam energy to a very small extent. To investigate the dependence of the dosimeter response to the radiation dose rate changes, eighteen crystals were exposed to dose rates of 100 cGy/ min, 200 cGy/min, 300 cGy/min, 400 cGy/min, 500 cGy/min and 600 cGy/min using the Elekta with an energy mode of 6 MV. The average of three crystals was considered for each dose rate, and the absorbed dose of the crystals versus dose rate was plotted in (Figure 5).
Figure 5: The variation of OSL response of the Al2O3 crystal versus the dose rate (MU/min). The absorbed dose for each crystal was 100 cGy and the data was normalized to the reading at 100 cGy/min. The error bars indicate ± σ
Twelve crystals were used to assess the repeatability of the dosimeters, where the crystals were subjected to a 100 cGy 3D dose irradiation of 6 mV photons. The repeatability curve of the dosimeters is shown in Figure. Since the values in (Figure 6) were normalized to the first reading, the repeatability variation was not significant and the maximum deviation was observed at the third reading (4%) (Figure. 6).
Figure 6: The reading repeatability of the Al2O3 dosimeter in the energy mode of 6 mV and dose of 100 cGy. The error bars indicate ± σ
Discussion
Several dosimeters (each with specific capabilities) are currently used in radiotherapy centers to measure the administered dose to the patient's body. High sensitivity to a wide range of energies, tissue-equivalency, repeatability of the dosimeter response, high stability, low fading, linear response to absorbed dose, and independence to irradiation rate are critical factors for a material to be used in dosimetry practice. Suitable properties of Al2O3 crystal at room temperature, high sensitivity (about 60 times more than LiF TLD-100), ability to store the signal for two years in a dark environment and/or low fading (less than 5% per year), re-reading possibility and sensitivity in a wide range of energies (10^(-7)-10 Gy) are the advantages of this dosimeter compared to TL samples (including TLDs) [27].
In this study, the dosimetric accuracy of the prototype Al2O3 crystal (developed in Malek-e-Ashtar University) in the megavoltage energy ranges was investigated and the relevant dosimetric characteristics were obtained. The excitation wavelength of the Al2O3 crystal was 260 nm, and the spectrum has three peaks at 308 nm, 375 nm and 595 nm. These results are in agreement with Hu and Akselord observations that showed TL has spectrum peaks at 420 nm and 330 nm [28].
Individual and group calibrations were carried out to assess the dosimetric characteristics of Al2 O3 crystals. The ECC values in individual calibration indicate that they are in the range of 0.996 ≤ ECC ≤ 1.003 (Table 1). These values are close to 1, indicating a low deviation of Al2O3 crystal readings.
In the group calibration curve, the best regression line was fitted to the points, wherein there was a linear relation between the crystal readings (Figure 3). Given the fitted line (y=0.0044x +1.37), it is possible to convert the Al2O3 crystals' reading to the absorbed dose. These results are in agreement with the results of Akselro study, that demonstrated the TL responses were linear and at the same rate in the range of 1 to 10 Gy [29]. On the other study, they investigated the highly sensitive thermoluminescent aniondeffective α-Al2O3 :C using exposure by 60C and a wavelength of 460 nm as the peak of OSL reader. Their results showed that the reponse of OSLs to irradiation at the range of 10-6 Gy– 102 Gy was linear similar to our readings [30].
Considering the Al2O3 absorbed dose variation against the changes in energy, the variations for the energy modes of 10 and 18 MV (relative to the energy of 6 mV as the reference) are 0.4% and 0.6%, respectively (Figure 4). In this light, there is no correlation between and/or sensitivity of crystal response to the energy of photon beam. The variation of the dosimeter OSL responses with respect to the changes in the dose rate was negligible (Figure 5). The mean deviation of OSLs responses to different dose rates in comparison to the reference dose rate (100 MU/min) is less than 0.4%. This indicates the independence of the dosimeter response to the dose rate changes. There is an agreement between these results and Yukihara observations, wherein the crystals showed a linear response to the dose ranges of 0.1 Gy-10 Gy [31].
The Al2O3 crystal exhibited acceptable repeatability, where the maximum deviation of the measurements was less than 0.5% (Figure 6). In this light, this prototype Al2O3 crystal bears appealing dosimetric characteristics such as independence of the dosimeter response to the energy of the radiation and the dose rate, as well as the linearity of response
Conclusion
The prototype Al2O3 crystal, evaluated in this work, exhibited interesting dosimetric characteristics such as linear and independent responses to dose rate and the energy of radiation. Therefore, this prototype could be a proper device for dosimetry practices in radiotherapy, personal dosimetry, Computer Radiography (CR) and Diagnostic Imaging
References
- Khan FM, Gibbons JP. Khan's the physics of radiation therapy. Lippincott Williams Wilkins. 2014.
- Redpath AT, Williams JR, Thwaites DI. Treatment planning of external beam therapy. InRadiotherapy phys pract. 1993:135-185. Oxf Univ Press Oxf.
- Baker J, Reinstein L, Gluckman G. Initial evaluation of a new radiochromic film for IMRT QA. InMEDICAL PHYSICS 2004:31, 1726-1726. STE 1 NO 1 2 HUNTINGT QUADRANG MELV NY 11747-4502 USA: AMER ASSOC PHYS MED AMER INST PHYS.
- Germer TA, Zwinkels JC, Tsai BK. Theoretical concepts in spectrophotometric measurements. InExperimental Methods Phys Sci 2014;46;11-66. Acad Press. 2014
- Todorovic M, Fischer M, Cremers F, Thom E, Schmidt R. Evaluation of GafChromic EBT prototype B for external beam dose verification. Med phys. 2006;33:1321-1328
- Cameron JR, Zimmerman D, Kenney G, Buch R, Bland R, Grant R. Thermoluminescent radiation dosimetry utilizing LiF. Health phys. 1964;10:25-29.
- Sasho Nikolovski S, Nikolovska L, Velevska M, Velev V. Thermoluminescent signal fading of encapsulated lif: Mg, Ti detectors in PTFE-Teflon registered trademark.
- Bøtter-Jensen L, McKeever SW, Wintle AG. Optically stimulated luminescence dosimetry. Elsevier. 2003.
- Hu B. Optically stimulated luminescence (OSL) and its applications in radiation therapy dosimetry.
- Yukihara EG, McKeever SW. Optically stimulated luminescence: fundamentals and applications. John Wiley Sons. 2011.
- WS McKeever S, Moscovitch M. Topics under Debate-On the advantages and disadvantages of optically stimulated luminescence dosimetry and thermoluminescence dosimetry. Radiat prot dosim. 2003;104:263-270.
- Benali AH, Ishak-Boushaki GM. Energy response of FD-7 RPL glass dosimeter compared with LiF Mg, Ti and Al2003: C dosimeters. J instrum. 2018;13:11015.
- Emfietzoglou D, Moscovitch M. Phenomenological study of light-induced effects in alpha-Al203: C. Radiat prot dosim. 1996;65:259-262.
- Yukihara EG, Yoshimura EM, Lindstrom TD, Ahmad S, Taylor KK, Mardirossian G. High-precision dosimetry for radiotherapy using the optically stimulated luminescence technique and thin Al2O3: C dosimeters. Phys Med Biol. 2005;50:5619.
- Akselrod MS, Kortov VS, Kravetsky DJ, Gotlib VI. Highly sensitive thermoluminescent anion-defect alpha-Al2O3: C single crystal detectors. Radiat prot dosim. 1990;33:119-122.
- Dhabekar B, Raja EA, Menon S, Rao TG, Kher RK, Bhatt BC. ESR, PL and TL studies of LiAlO2: Mn/Ce phosphor. Radiat meas. 2008;43:291-294.
- Temuujin J, Jadambaa T, Mackenzie KJ, Angerer P, Porte F, Riley F. Thermal formation of corundum from aluminium hydroxides prepared from various aluminium salts. Bull Mater Sci. 2000;23:301-304.
- Han JH, Martin ST. An aerosol chemical reactor for coating metal oxide particles with (NH4) SO4-H2SO4-H2O. 1. New particle formation. Aerosol Sci Technol. 2001;34:363-372.
[CrossRef]
- Hernandez T, Bautista MC. The role of the synthesis route to obtain densified TiO2-doped alumina ceramics. J Eur Ceram Soc. 2005;25:663-672.
- Janbey A, Pati RK, Tahir S, Pramanik P. A new chemical route for the synthesis of nano-crystalline α-Al2O3 powder. J Eur Ceram Soc. 2001;21:2285-2289.
- Patil KC, Aruna ST, Mimani T. Combustion synthesis: an update. Curr opin solid state mater sci. 2002;6:507-512.
- Ianoş R, Lazău I, Păcurariu C. The influence of combustion synthesis conditions on the α-Al 2 O 3 powder preparation. J mater sci. 2009;44:1016-1023.
- McKittrick J, Shea LE, Bacalski CF, Bosze EJ. The influence of processing parameters on luminescent oxides produced by combustion synthesis. Displays. 1999;19:169-172.
- Zazouli MA, Kalankesh LR. Removal of precursors and disinfection by-products (DBPs) by membrane filtration from water; a review. J Environ Health Sci Eng. 2017;15:1.
- Colyott LE, Akselrod MS, McKeever SW. Phototransferred thermoluminescence in alpha-Al2O3: C Radiat prot dosim. 1996;65:263-266.
- Whitley VH, McKeever SW. Photoionization of deep centers in Al2O3. J appl phys. 2000;87:249-256.
- Williams JR, Thwaites DI. Radiotherapy physics in practice. 2000.
- Akselrod MS. Dosimetric properties of Al 2 O 3 crystals for optically and thermally stimulated luminescent dosimetry.
- Akselrod MS, Bøtter-Jensen L, McKeever SW. Optically stimulated luminescence and its use in medical dosimetry. Radiat Meas. 2006;41:78-99.
- Akselrod MS, Kortov VS, Kravetsky DJ, Gotlib VI. Highly sensitive thermoluminescent anion-defective alpha-Al203: C single crystal detectors. Radiat Prot Dosim. 1990;32:15-20.
- Yukihara EG, Mardirossian G, Mirzasadeghi M, Guduru S, Ahmad S. Evaluation of optically stimulated luminescence (OSL) dosimeters for passive dosimetry of high‐energy photon and electron beams in radiotherapy. Med phys. 2008;35:260-269.